Sectioning, Mounting, Grinding, Surface Preparation, Technique for Polishing and Etching of Materials (including Electropolishing, tint etching etc.)
Metallography is the study of the microstructure of metals and alloys using microscopy. It is the art and science of preparing, interpreting, and analyzing microstructures in materials to understand materials behavior and performance better. The method is used to evaluate metallic materials in various industries, including the aerospace industry, the automotive industry, and parts of the construction industry. It is also used for process control, including examining defects that appear in finished or partly finished products, as well as the studies of parts that have failed during service. It is an essential branch of the Metallurgical Engineering.
A well-prepared metallographic specimen is
- A representative sample
- Sectioned, ground, and polished to minimize disturbed or flowed surface metal caused by mechanical deformation and thus to allow the true microstructure to be revealed by etching.
- Free from polishing scratches and pits and liquid staining
- Flat enough to permit examination by optical microscope or SEM.
Various steps involved in the specimen preparation and the significance of each step
Many steps in the preparation of metallographic specimens described here are also applicable in other types of metallographic studies, such as electron microscopy, micro-hardness testing, quantitative measurement of constituents of structures, and electron microprobe analysis. Preparation of metallographic specimens generally requires five primary operations:
- Sectioning
- Mounting (which is necessary when the sample cannot be held properly due to its shape and/or size while polishing)
- Grinding
- Polishing
- Etching
These operations are listed below in the order they are performed.
1 Sectioning
Separate test pieces or coupons attached to castings or forgings should be designed so that a minimum of sectioning is required for producing metallographic specimens. Sectioning becomes necessary when studying parts that have failed in service, where a specimen must be taken from a large block of material. Therefore, metallographic studies of such samples often involve more than one sectioning operation.
Many metallographic studies require more than one specimen. For example, a study of deformation in wrought metals usually requires two sections- one perpendicular to, and the other parallel to, the central axis of the direction of deformation. Failed parts may best be studied by selecting a specimen that intersects the origin of the failure if the origin can be identified on the surface. Depending on the type of failure, it may be necessary to take several specimens from the area of failure and adjacent areas.
2 Mounting of Specimens
The primary purpose of mounting is to make it convenient to handle specimens of arbitrary shape and/or small sizes during various steps of metallographic sample preparation and examination. A secondary purpose is to protect and preserve extreme edges or surface defects during metallographic preparation. Specimens may also require mounting to accommodate various types of automatic devices used in metallographic laboratories or to facilitate placement on the microscope stage. An additional benefit of mounting is identifying the sample (name, alloy number, or laboratory code number) without damaging the specimen.
2.1 Compression mounting:
It is the most common mounting method, which involves molding around the metallographic specimen by heat and pressure using molding materials such as Bakelite, Diallyl Phthalate resins, and acrylic resins. Bakelite and Diallyl phthalate are thermosetting, and acrylic resins are thermoplastic.
Not all materials or specimens can be mounted in thermosetting or thermoplastic mounting. The heating cycle may cause changes in the microstructure, or the pressure may cause delicate specimens to collapse or deform. The size of the selected specimen may be too large to be accepted by the available mold sizes. These difficulties are usually overcome by cold mounting.
Cold Mounting requires no pressure and little heat and is a means of mounting large specimens more rapidly than possible by compression mounting. Epoxy resins are the most widely used cold mounting materials. They are hard and adhere tenaciously to most metallurgical, mineral, and ceramic specimens.
3 Grinding
Grinding is the most important operation in specimen preparation. During grinding, the operator has the opportunity to minimize mechanical surface damage that subsequent polishing operations can remove. Even if sectioning is done carelessly, resulting in a severely damaged surface, the damage can be eliminated by prolonged grinding. However, prolonged grinding should be avoided since it might lead to excess heating or surface damage.
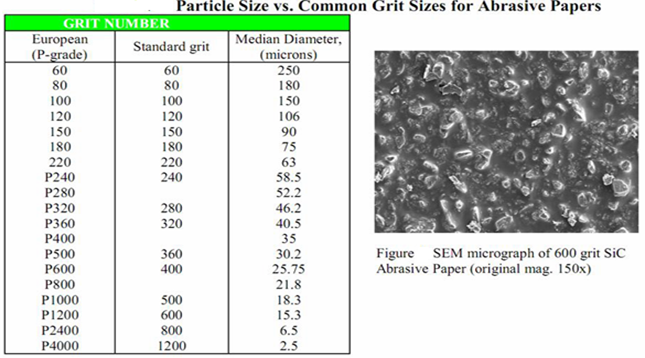
Grinding is accomplished by abrading the specimen surface through a sequence of operations using progressively finer abrasive grits. Grit sizes ranging from 60 mesh to 150 mesh are usually regarded as coarse abrasives, and grit sizes ranging from 180 mesh to 600 mesh as fine abrasives. Grinding should commence with the coarse grit size for making an initial flat surface and remove the effects of sectioning. Hack-sawed, band-sawed, or rough surfaces usually require abrasive grit sizes in the 80 to 150 mesh range. Grinding should be done sequentially, from the coarser to the finer. A satisfactory grinding sequence might involve 180, 240, 400, and 600 mesh grit sizes. Grinding aims to remove the oxide layer's damaged layer or uneven surfaces that might have formed during the last sectioning operation. However, the depth of cold-worked metal is roughly inversely proportional to the hardness of the specimen and maybe 10 to 50 times the abrasive particle's penetration depth.
To ensure the complete elimination of the previous grinding scratches found by visual inspection, the direction of grinding must be changed by 90o from the one stage of grinding to the next stage. In addition, microscopic examination of the various ground surfaces during the grinding sequence may be worthwhile in evaluating the effect of grinding. Each ground surface should have scratches that are clean-cut and uniform in size, with no evidence of previous grinding scratches. Cleaning before going to the next stage of grinding is always helpful.
3.1 Grinding Mediums
Grinding media are silicon carbide (SiC), aluminum oxide (Al2O3), emery (Al2O3-Fe3O4), and diamond particles. All except diamonds are generally bonded to paper or cloth backing material of various weights in the form of sheets, disks, and belts of various sizes. The abrasives may also be used in powder form by charging the grinding surfaces with loose abrasive particles.
Automatic grinding, as the name implies, is done without hand assistance. All automatic grinding devices use lap surfaces on which paper-based disks are placed or abrasive powder is charged. The lap is either a rotating or a vibrating disk, where the latter is described as vibratory grinding.
4 Polishing
Polishing is the final step in producing a flat, scratch-free surface and mirror-like appearance. Such a surface is necessary for subsequent accurate metallographic interpretation, both qualitative and quantitative. The polishing technique should not introduce extraneous structure, such as disturbed metal, pitting, dragging out of inclusions, and staining.
4.1 Mechanical Polishing
Mechanical polishing is frequently used to describe the final polishing procedures involving cloth-covered laps and suitable polishing abrasives, such as Al2O3 slurry. The laps have either a rotating or a vibrating motion, and the specimens are held by hand, held mechanically, or merely confined within the polishing area. Polishing should be done in a relatively dust-free area, preferably removed from the area for sectioning, mounting, and rough grinding. Any contamination of a polishing lap by abrasive particles carried over from preceding operations or by dust, dirt, or other foreign matter in the air cannot be tolerated. Carryover because of improper cleaning between final polishing steps is another prime source of contamination. It is just as crucial for the operator to wash his/her hands meticulously as it is for him/her to remove all traces of polishing abrasive from the specimen before proceeding to the next finer polishing operation.
4.2 Electrolytic Polishing
Even with the most careful mechanical polishing, some disturbed metal, even a very small amount, will remain after the preparation of a metallographic specimen. This is no problem if the specimen is to be etched for structural investigation because etching is usually sufficient to remove the slight layer of disturbed metal. However, electrolytic or chemical polishing is preferred if the specimen is to be examined in the polished condition or if no surface disturbance can be tolerated. The basic principle involved in this technique is anodic dissolution of the sample's surface which results in a leveling and brightening of its surface.
Electro polishing does not disturb any metal on the specimen surface and is ideally suited for the metallographic preparation of soft metals, most single-phase alloys, and alloys that work harden readily. The disadvantages of electro-polishing include preferential attack in multiphase alloys caused by differences in electrical potential between phases. Proper choice of electrolyte and operating conditions will minimize these disadvantages.
Metals, especially stainless steel, and other alloys can have their surfaces treated using an electrochemical process called electropolishing. This procedure is intended to leave a metal surface with a smooth, polished, and frequently visually appealing finish by removing a predetermined amount of material. Electropolishing is frequently used in a variety of industries where a superior surface finish is essential, such as the medical, pharmaceutical, aerospace, and electronics sectors.
4.2.1 Principle
The foundation of electropolishing is the anodic dissolution principle, which involves using an electrolytic cell to selectively remove metal from a workpiece's surface. Electropolishing produces a smoother and more reflective finish by uniformly removing material from the entire surface, in contrast to traditional polishing techniques.
4.2.2 Equipment and setup
Electrolyte bath
An appropriate electrolyte solution is made, usually with certain additives and acids. Mixtures of sulfuric acid, phosphoric acid, and other chemicals are common electrolytes.Cathode and anode
The anode of the electropolished workpiece is the same as the cathode, which is also submerged in the electrolyte bath and is typically composed of stainless steel. The anode and cathode are connected by an electric current.Power supply
Electric current is applied between the anode and cathode using a direct current (DC) power supply.
4.2.3 Advantages of electropolishing-
- Improved surface finishing
Surface roughness is reduced by electropolishing, which creates a smooth, mirror-like surface. - Deburring and smoothing
The removal of burrs and sharp edges improves component safety. - Corrosion resistance
By passivating the metal surface, the procedure increases its corrosion resistance. - Micro finish improvement
Surface finishes as fine as microinches can be obtained by electropolishing.
4.2.4 Applications:
- Medical devices
Surgical instruments and implants - Aerospace
Components for aircrafts and spacecrafts - Automotive
Engine components and decorative parts - Electronic
Semiconductor and electronic components
Enhancing the surface qualities of metal components through electropolishing offers several advantages, including improved corrosion resistance, functional performance, and aesthetic appeal. Its extensive application in a variety of industries attests to its significance for engineering and manufacturing procedures.
5 Etching
Metallographic etching is used to reveal the structural characteristics of a metal. This is essential since these structural characteristics are not visible in the polished mirror-like surface of the metal. It can be used for phase identification, dislocation density calculation (etch pitting), and orientation studies. The principle of etching multiphase alloys is based on the preferential attack (different rates of electrochemical dissolution of phases in the etchant) or preferential staining of one or more phases because of differences in chemical composition and grain orientation. Before being etched, a specimen should be inspected for polishing defects, such as scratches, pits, relief polish, comet tails, pulled-out inclusions, and voids.
5.1 Chemical Etching
Chemical etching is accomplished by immersing the specimen in (or swabbing it with) a suitable etchant until the required structure is revealed. Etching is done in Petri dishes or in other suitable containers with loose covers to prevent excessive evaporation of the solvent, particularly alcohol solutions. Glass containers can be used for all etchants except hydrofluoric acid solutions, where the container should be made of polyethylene or other suitable material. Using tongs or other convenient handling devices, the surface of the specimen is immersed in the etchant with some agitation to ensure that fresh etchant is in contact with the specimen all the time. Most metals lose their bright appearance during etching, indicating that etching is taking place. With practice, one can ascertain the completion of etching by the degree of dullness of the surface. If the etching procedure calls for swabbing, the surface of the specimen can be swabbed with cotton saturated with the etchant, or the specimen can be immersed and swabbed while in the solution. When etching is complete, the specimen is rinsed in running water and then in alcohol, followed by drying in a stream of warm air (hand dryer). After etching, the specimen surface is observed under the optical microscope to study its microstructure. Care should be taken while etching so that the hand is not affected by the etching.
5.2 Tint Etching:
Tint etchants are designed to color etch a wide range of metals and alloys, including lead, tungsten, molybdenum, zinc, steel, stainless steel, nickel base alloys, and copper base alloys. Tint etching of titanium and aluminium alloys has yielded varying degrees of success. The most widely used tint etchant is the one created by Klemm, which colors ferrite in steels, reveals burning or overheating in steels, and develops the grain structure of lead, tin, and zinc in addition to copper and many copper alloys.
Chemical balancing is used in satisfactory tint, or stain, etchants to create a stable film on the specimen surface. In contrast, the corrosion products created during etching are redissolved into the etchant in regular chemical etchants. Anodic, cathodic, and complex systems are the different classifications for tint etchants based on the type of film precipitation. Etching is a regulated process of corrosion that relies on the electrolytic interaction of surfaces with varying potentials. Between grains of different orientations, between grain boundaries and interiors, between impurity phases and the matrix, or at concentration gradients in single-phase alloys, there may be a difference between pure metals and single-phase alloys. A potential also exists between the different phases that are present in multiphase alloys.
When chemical etchants are used, these possible variations change the rate of attack, exposing the microstructure. One phase of an alloy with two phases has a higher potential than the other. The more electronegative (cathodic) phase is not significantly attacked during etching, but the more electropositive (anodic) phase is. The potential difference between two phases is larger than the potential differences found in alloys with only one phase. As a result, alloys containing two or more phases etch faster than alloys and metals with just one phase.
Typically, anodic, or cathodic phases are colored by tint etchants. Tint etchants for steels that are selective to the phases that are typically cathodic have been developed with some degree of success. On the other hand, most tint etchants color the anodic phases. Typically, acidic solutions with alcohol or water as the solvent are used as tint etchants. They are designed to cover the surface of the specimen with a thin layer, usually 40–500 nm thick, of an oxide, sulphide, complex molybdate, elemental selenium, or chromate.
Interference is the same process that creates color as vacuum deposition or heat tinting. Tint etchants are only effective when immersed; they cannot be used to create films by swabbing. Potentials applied externally are not employed. Colors are controlled by the thickness of the film. When viewed with white light, interference produces colors in the typical order of yellow, red, violet, blue, and green as the film thickness increases. In anodic systems, the film only forms on top of the anodic phase, but its thickness varies according to the phase's crystallographic orientation. Therefore, the etch time needs to be kept constant to produce the same color every time. Usually, this is done by timing the etch and observing the sample's macroscopic color while it is being stained. Tint etchants, created by Beraha, leave a thin layer of sulphide on a variety of metals, including copper, copper alloys, nickel base alloys, steels, and cast irons.
Sodium metabisulfite (Na2S2O5), potassium metabisulfite (K2S2O5), and sodium thiosulfate ((Na2S2O3· 5H2O) are common ingredients in tint etchants. These are typically used to color anodic phases and are used with water as the solvent. Hydrochloric acid is added to tint more metals that are resistant to acid. These substances are found in tint etchants, which result in sulphide films. Sulphur dioxide and hydrogen sulphide odors are noticeable when in use. Etching ought to be done under a hood, even though this is just a small annoyance.