DSB-SC Modulation
Theory
Double Sideband Suppressed Carrier (DSB-SC) modulation is a type of amplitude modulation where only the sidebands are transmitted, and the carrier signal is suppressed. This method is more power-efficient compared to standard amplitude modulation (AM), as it eliminates the carrier, which does not carry useful information.
Mathematical Representation
The DSB-SC modulated signal \( S(t) \) can be expressed as:
\( S(t) = A_c m(t) \cos(2\pi f_c t) \)
Where:
- \( A_c \) is the amplitude of the carrier signal.
- \( m(t) \) is the baseband (modulating) signal.
- \( f_c \) is the frequency of the carrier signal.
In DSB-SC modulation, the carrier signal \( \cos(2\pi f_c t) \) is multiplied by the modulating signal \( m(t) \), resulting in the modulated signal \( S(t) \). The key characteristic of DSB-SC is that the carrier \( A_c \cos(2\pi f_c t) \) is not transmitted. Instead, only the sidebands generated by the multiplication of \( m(t) \) and \( \cos(2\pi f_c t) \) are transmitted.
Block Diagram
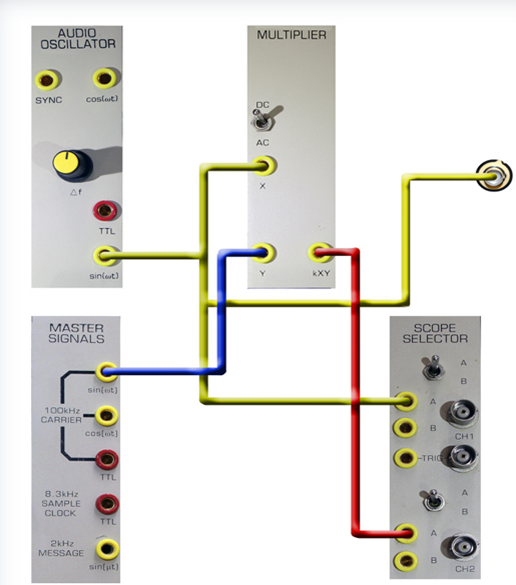
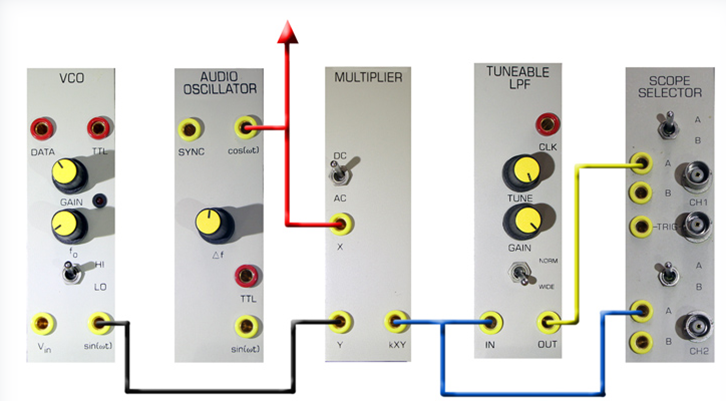
Figure 1
Procedures :
1. Patch up the arrangement of
Figure 3.
2. Use the FREQUENCY COUNTER to
set the AUDIO OSCILLATOR to about 1 kHz.
3. Measure and record the
amplitudes A and B of the message and carrier signals at the inputs
to the MULTIPLIER.
4.
DSBSC = k A.cosμt B.cosωt .
5. Now peak to peak amplitude of
the DSBSC signal will be 2*K.A.B volts.
6. Here 'k' is a scaling factor,
a property of the MULTIPLIER. One of the purposes of this experiment
is to determine the magnitude of this parameter.
7. Measure the peak-to-peak
amplitude of the DSBSC. Since you have measured both A and B
already, you have now obtained the magnitude of the MULTIPLIER scale
factor 'k'; thus:
k = (dsbsc peak-to-peak) / (2 A B); Note that 'k' is not a
dimensionless quantity
8. For showing the fine detail
inside the DSBSC by Oscilloscope it is necessary that ‘µ’ be a
sub-multiple of ‘ω’
9. Insert a BUFFER AMPLIFIER in
one or other of the paths to the MULTIPLIER, and increase the input
amplitude of this signal until overload occurs.
10. Now if you pass the DSB-SC
signal thru the 60 KHz ‘LOWPASS LPF’ module then it result in no
output as message frequency itself is 1 KHz. So, upper and lower
side bands are 99 KHz and 101 KHz respectively
11. Now we’ll use VCO to
generate carrier signal at our convenience.
12. Adjust the VCO frequency to
about 10 kHz .
13. Set the AUDIO OSCILLATOR to
about 1 kHz.
14. Set the front panel GAIN
control of the TUNEABLE LPF so that the gain through the filter is
unity.
15. Analysis predicts that the
DSBSC is centred on 10 kHz, with lower and upper side frequencies at
9.0 kHz and 11.0 kHz respectively. Both side frequencies should fit
well within the pass-band of the TUNEABLE LPF, when it is tuned to
its widest pass-band, and so the shape of the DSBSC should not be
altered.
16. Set the front panel toggle
switch on the TUNEABLE LPF to WIDE, and the front panel TUNE knob
fully clockwise. This should put the pass-band edge above 10 kHz.
The pass-band edge (sometimes called the ‘corner frequency’) of the
filter can be determined by connecting the output from the TTL CLK
socket to the FREQUENCY COUNTER. It is given by dividing the counter
readout by 360 (in the ‘NORMAL’ mode the dividing factor is 880).
17. Note that the pass-band GAIN
of the TUNEABLE LPF is adjustable from the front panel. Adjust it
until the output has a similar amplitude to the DSBSC from the
MULTIPLIER (it will have the same shape). Record the width of the
pass-band of the TUNEABLE LPF under these conditions.
18. Assuming the last Task was
performed successfully this confirms that the DSBSC lies below the
pass-band edge of the TUNEABLE LPF at its widest. You will now use
the TUNEABLE LPF to determine the sideband locations.
19. The cut-off frequency of
this LOWPASS FILTER can be varied using the TUNE control. Two
frequency ranges, WIDE and NORMAL, can be selected by a front panel
switch. The GAIN control allows signal amplitudes to be varied if
required.
20. NORMAL range provides
more precise control over the lower audio band, used for
telecommunications message channels. The WIDE range expands
the filter’s range to above 10 kHz.
21. Lower the filter pass band
edge until there is a just-noticeable change to the DSBSC output.
Record the filter pass band edge as fA. You have located the upper
edge of the DSBSC at (w + μ) rad/s.
22. Lower the filter pass-band
edge further until there is only a sine wave output. You have
isolated the component on (ω - μ) rad/s. Lower the filter pass band
edge still further until the amplitude of this sine wave just starts
to reduce. Record the filter pass band edge as fB.
DSB-SC Demodulation
DSBSC Demodulation :
Recovering the message signal from the demodulated signal is
performed coherently. That is, the demodulated signal is multiplied
by a high-frequency sinusoid in perfect synchronization (in phase
and frequency) with the incoming carrier.
This requirement poses a challenge on the design of the demodulator
circuit, as it would then require a part for carrier-recovery.
Failing to accomplish perfect synchronization will result in phase
mismatch or frequency mismatch, leading to some form of distortion
in the recovered signal.
Multiplying the modulated signal with a local carrier will produce a
baseband signal as well as a signal modulated at double the carrier
frequency. Therefore, a LPF is needed at the far end of the
demodulator to recover the baseband signal .
DSBSC Demodulation Block Diagram
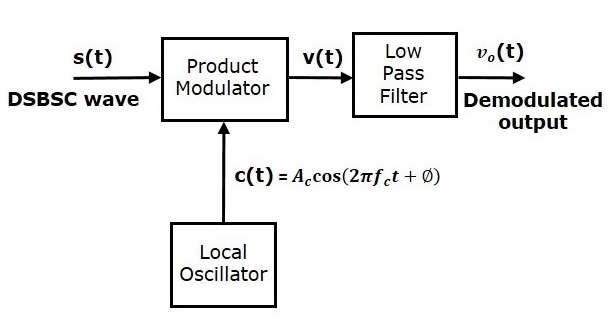
Fig. DSB-SC Demodulation Block Diagram
Procedures :
1. Use the same carrier signal used in DSBSC modulation, multiplier and a Tunable LPF to demodulate the DSBSC generated during DSBSC modulation.
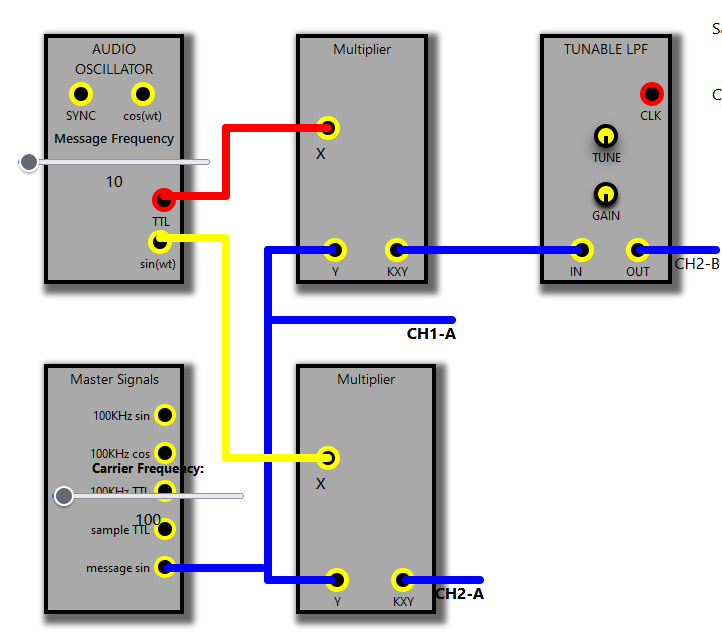
Fig. The TIMS Model of The Block Diagram of DSB-SC Demodulation
2. Switch the Scope Selector to
CH1-A and CH2-B.
3. Observe the signal in time and
frequency domains before and after the LPF simultaneously.
4. Vary the cutoff frequency of
the LPF, and find the range of acceptable values for best recovery
of the message.
5. Plot, in time, the best
recovered signal you can obtain in your lab sheets.
6. Increase the cutoff frequency
of the LPF beyond the range of good recovery.